EXECUTION STAGE 1/2021
Synthesis and characterization of cellulose-based derivatives: the future matrix for N-heterocyclic compounds incorporation
• Selective oxidation reactions of the primary OH groups for different cellulosic sorts were performed by processes that are developed in our laboratory, the structure of oxidized materials being confirmed by FTIR, 13C-NMR and X rays, morphology by scanning electron microscopy (SEM). These materials represent the basis that will be used later for the preparation of proton conducting membranes in the next steps.
• The introduction of a considerably larger number of carboxylic groups into the structural unit of cellulose was made possible by its functionalization with citric acid, a reaction performed at high temperature.
• An ecological alternative was presented for the production of nanocrystalline cellulose from agricultural waste, namely hemp stems resulting from the harvesting process.
• All the indicators provided in the “project implementation plan” were completed: 3 papers submitted for publication (completed 5), 2 oral presentations and 4 posters (completed 3 oral presentations and 4 posters).
Activity 1.1. Preparation and characterization of carboxylated cellulosic substrates by the TEMPO oxidation method
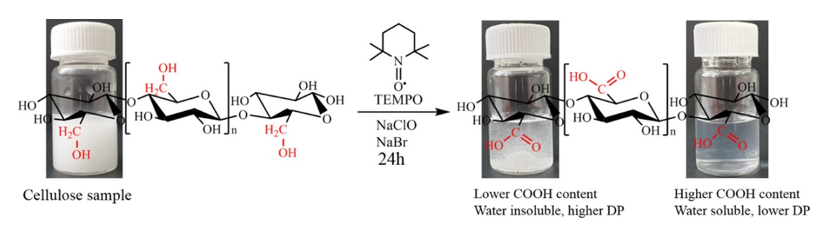
Fig. 1. Pattern of cellulose oxidation reaction in the presence of TEMPO, NaClO, NaBr
The oxidation products showed almost homogeneous structures of poly-β-D-(1→4)-sodium glucuronate or sodium salt of celuronic acid (CUA) consisting only of D-glucuronosyl units. Therefore, the primary hydroxyl groups in the cellulose structure can be converted entirely and selectively into carboxylate groups (carboxylate groups in the form of sodium salt) by TEMPO-mediated oxidation. It has been confirmed that C6-carboxylic groups cannot be generated only by the TEMPO radical, requiring the presence of NaBrO and/or NaClO in the system at pH 10 (Figure 2).
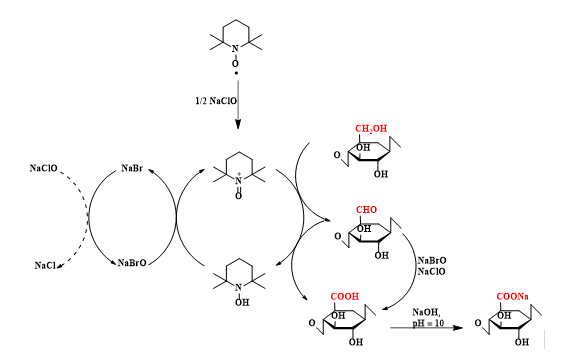
Fig. 2. Regioselective oxidation of hydroxyl groups located at C6 from the structure of cellulose to C6-carboxylic groups by means of the oxidation system TEMPO/NaBr/NaClO in water, at pH 10 - 11
Table 1 shows yields, degrees of oxidation (OD), degrees of polymerization (GP), zeta potential, and weight loss for oxidized cellulose fractions.
Table 1. Yields, degrees of oxidation, degrees of polymerization, zeta potentials and weight loss for various samples of oxidized cellulose
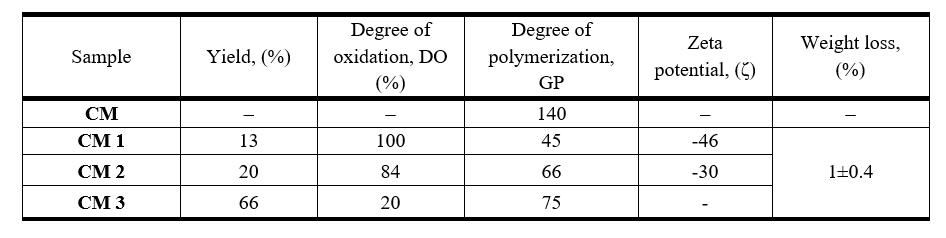
The samples were characterized from several points of view:
- Nuclear magnetic resonance (13C-RMN)
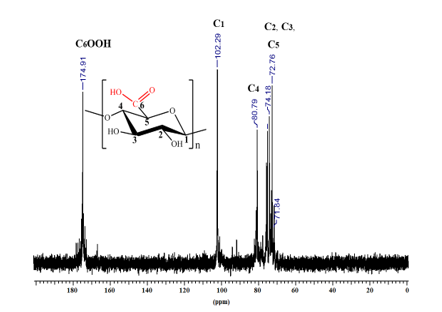
Fig. 3. 13C-NMR spectrum (in D2O) of the oxidized cellulose sample (CM 1, GO = 100%)
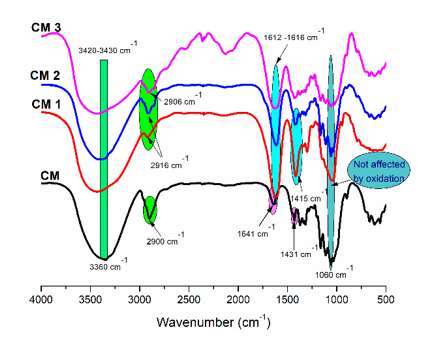
Fig. 4. FTIR spectra of non-oxidized cellulose (CM) and oxidized samples (CM 1, CM 2 and CM 3)
- Scanning electron microscopy (SEM)
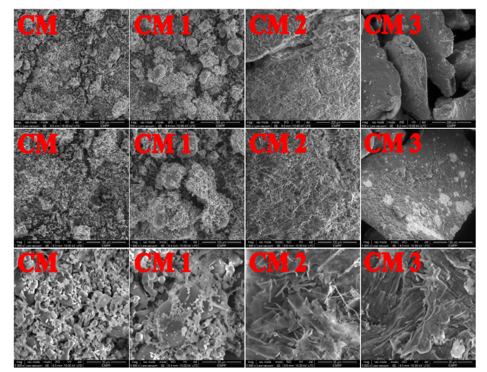
Fig. 5. SEM micrographs of microcrystalline cellulose (CM) before oxidation and its oxidized fractions (CM 1, CM 2 and CM 3), magnified 500, 1000 and 5000 times, respectively
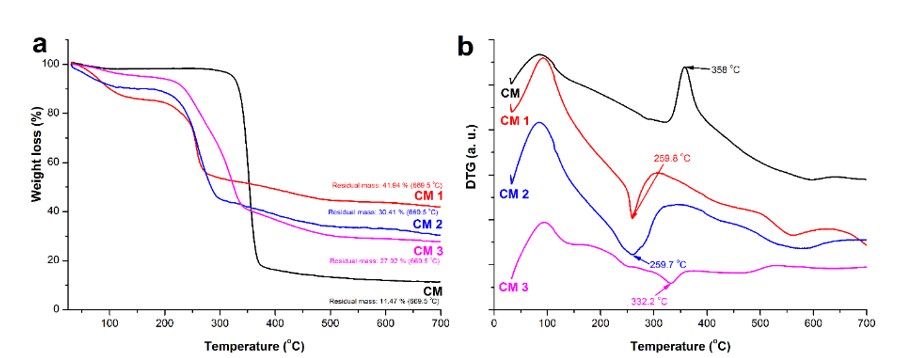
Fig. 6. Thermogravimetric curves (a) and corresponding thermogravimetric derivatives of cellulose samples before and after oxidation (b)
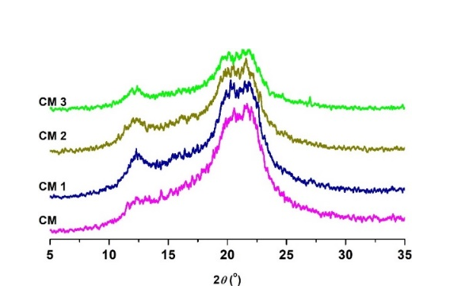
Fig. 7. X-ray diffractograms of cellulosic samples before and after oxidation
Activity 1.2. Preparation of carboxylated cellulosic substrates by functionalizing cellulosic sorts with citric acid
This activity aimed to prepare citric acid modified cellulose (CA) in order to obtain a high content of carboxylic groups on the cellulose surface. The chemical reaction that takes place on the surface of cellulose fibrils is described in Figure 8.
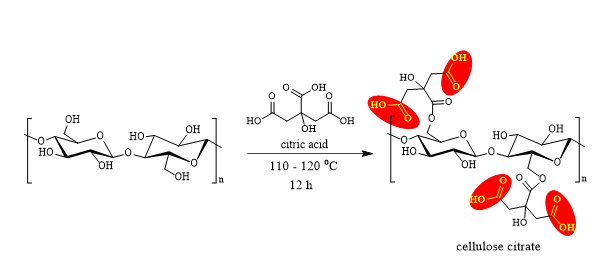
Fig. 8. Chemical reaction between cellulose and citric acid
A sample of Cotton Linters was chosen for the preparation of carboxylated cellulose substrates. Final samples were characterized from several points of view:
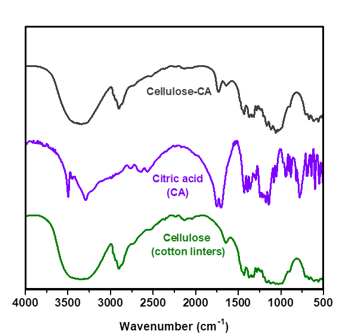
Fig. 9. FTIR spectra for cellulose sample (Cotton Linters), citric acid (CA) and cellulose modified with citric acid (Cellulose-CA)
- Dynamic light scattering method (DLS)
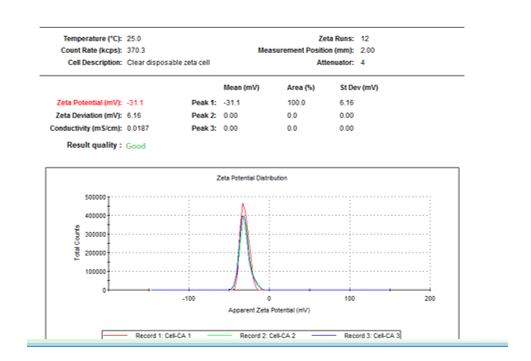
Fig. 10. Value of zeta potential for cellulose functionalized with citric acid
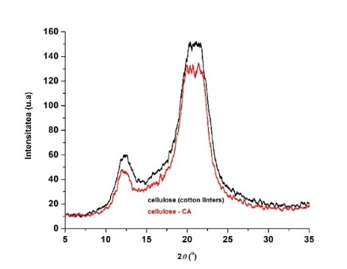
Fig. 11. X-ray diffractograms of cellulosic samples: initial cellulose and cellulose functionalized with citric acid
Activity 1.3. Preparation of nanocellulose from agricultural waste
For this research activity, we turned our attention to the preparation of nanocrystalline cellulose (CNC) starting from plant residues from hemp stems. This process involved in the first phase the separation of lignin and hemicellulose cellulose from plant residues, then the cellulose obtained was subjected to specific treatment processes in order to obtain nanocellulose. Obtaining and characterizing nanocellulose can have a significant impact on the research we undertake, being well known the benefits of using nanocellulose as an additive in composite materials, hydrogels, due to the remarkable properties that this compound transmits to final materials.
The nanocellulose extraction process consisted of several stages:
- Treatment of hemp with ethanol
- Hemp treatment with sodium hydroxide
- Treatment of hemp with sodium hypochlorite
- Treat hemp with hydrogen peroxide
Figure 12 describes in detail the transition from raw material (hemp stalks) to cellulose following chemical treatments.
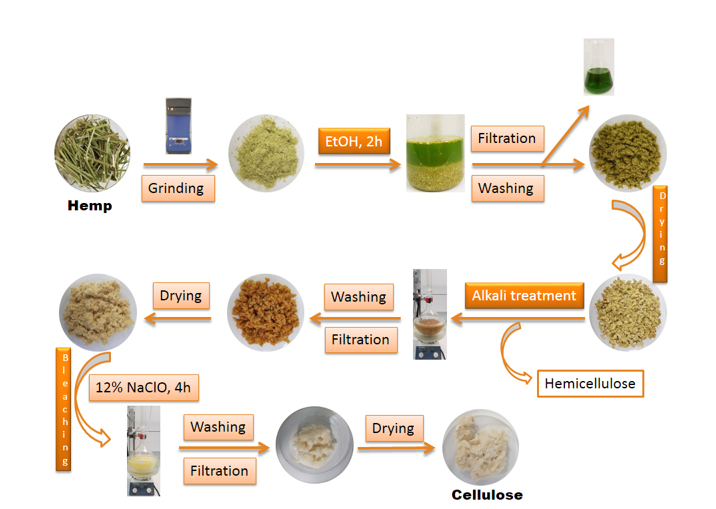
Fig. 12. Synthesis of nanocellulose from plant raw materials, from hemp stems
Oxidation of 2,2,6,6-tetramethylpiperidine-N-oxyl-mediated cellulose (TEMPO) - Figure 13 shows schematically the process of the cellulose oxidation reaction.
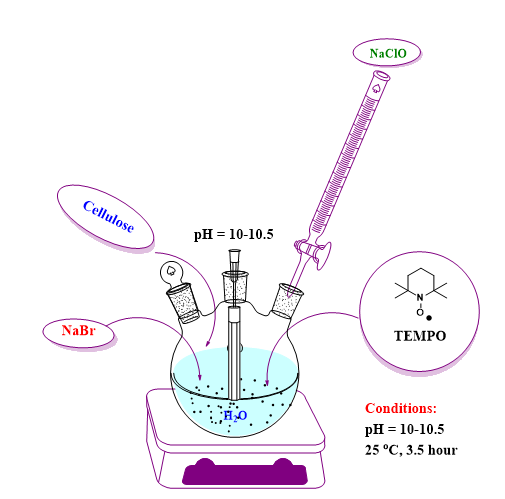
Fig. 13. Schematic representation of the working method for obtaining oxidized cellulose
The mechanism of the TEMPO radical-mediated oxidation reaction is shown in Figure 14. NaClO, used as the primary oxidant, first oxidizes the TEMPO radical to the N-oxoammonium or TEMPO+ structure, which then oxidizes the primary hydroxyl groups in cellulose to carboxylic groups, through aldehyde groups (C-6). N-hydroxy-TEMPO or its reduced form is oxidized by sodium hypobromite (NaBrO), formed in the reaction medium in the presence of sodium bromide (NaBr) and sodium hypochlorite (NaClO). Thus, TEMPO and NaBr behave as catalysts and only NaClO is consumed during oxidation. Because carboxylic groups are formed by the oxidation reaction, maintaining the reaction medium at pH = 10 is done with a small amount of 0.5 M NaOH solution. Some of the aldehyde groups are oxidized directly to carboxylic groups with NaClO and/or NaBrO.
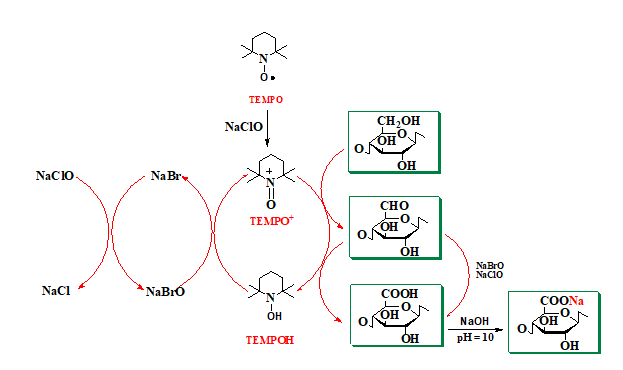
Fig. 14. Regioselective oxidation of primary alcohol groups from cellulose to carboxylic groups via the TEMPO/NaBr/NaClO oxidation system, at pH 10
The samples were characterized from several points of view:
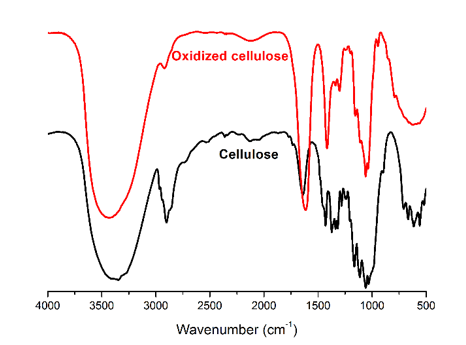
Fig. 15. FTIR spectra of cellulose and oxidized cellulose via the TEMPO/NaBr/NaClO oxidation system
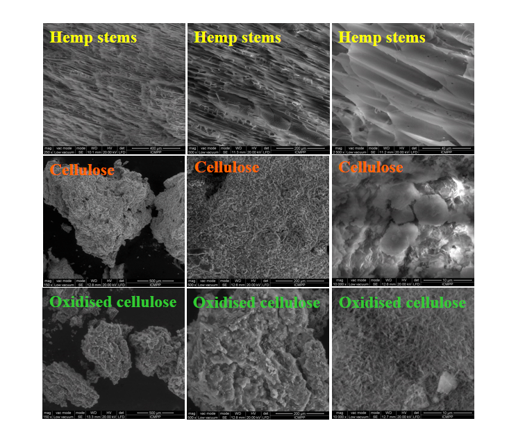
Fig. 16. SEM images of hemp stems, cellulose and oxidised cellulose via the TEMPO/NaBr/NaClO oxidation system
- Transmission electron microscopy (TEM)
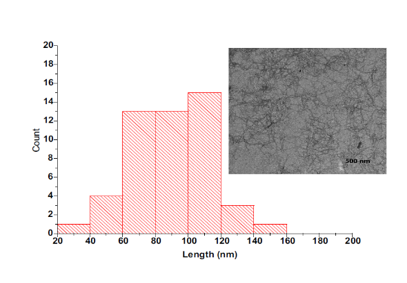
Fig. 17. Cellulose fiber length distribution and TEM image of the oxidized cellulose sample
EXECUTION STAGE 2/2022
Fabrication of hybrid composite membranes from cellulose-based matrices and different N-heterocyclic compounds
Fuel cells are currently the subject of a global research trend due to the rising demand for such devices. Even though the fundamental concept was developed more than 150 years ago by Sir W.R. Grove, fuel cell (FC) technology might be one of the options for innovative and sustainable energy generation. The electrolyte membrane is a critical component for fuel cell performance and serves as a separator between the anode and cathode.
Any material can be used as a proton exchange membrane if several conditions are concomitantly satisfied:
i) high proton conductivity
ii) good thermal and chemical stability
iii) outstanding tensile strength
iv) electron insulator
Main cellulosic materials used in this step:
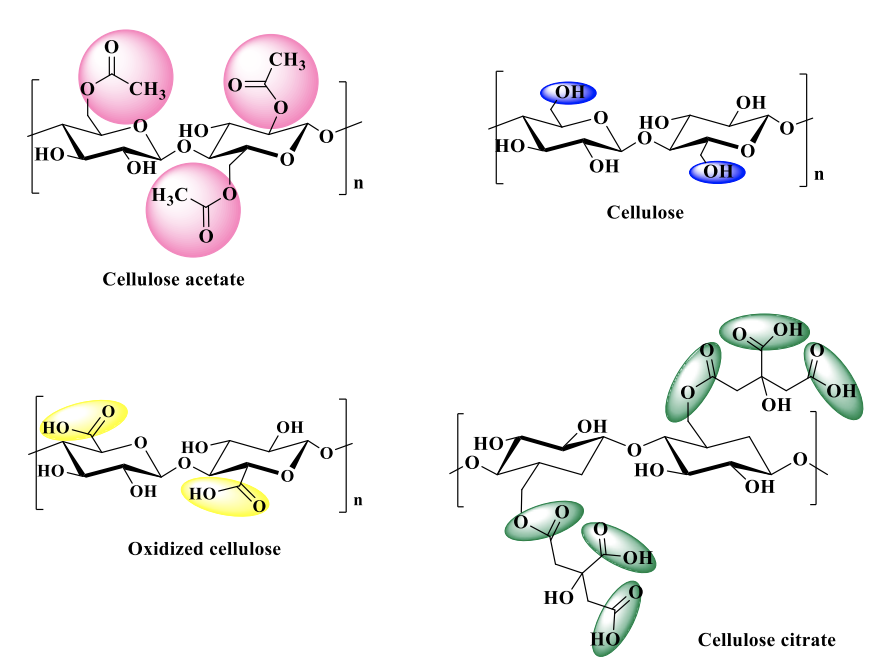
Fig. 1. Cellulose and its derivatives structures used in the execution stage 2/2022
Main heterocyclic compounds used in the execution stage 2/2022:
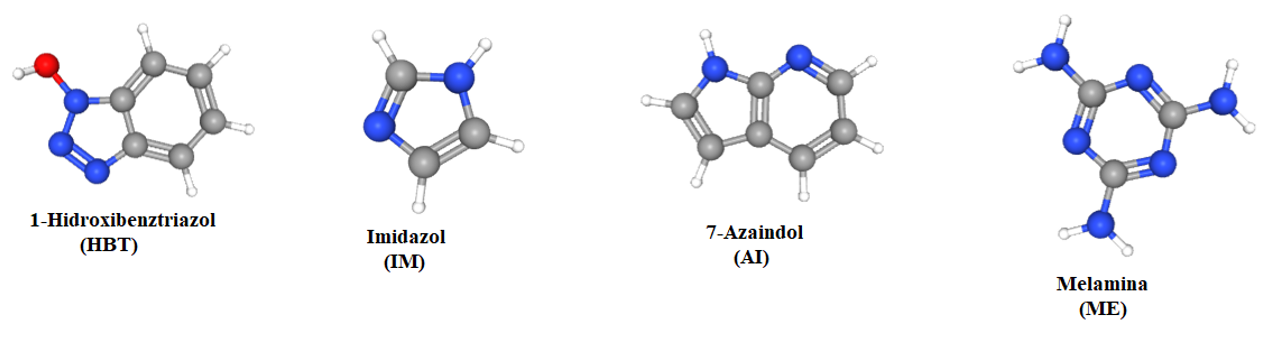
Fig. 2. Molecular structures of heterocyclic compounds used in the execution stage 2/2022
The results of molecular docking are highlighted in Figure 3, showing the best receptor-ligand complexation poses for each case (i.e. for cellulose receptor (Figure 3a) and for oxidized-cellulose receptor (Figure 3b)).
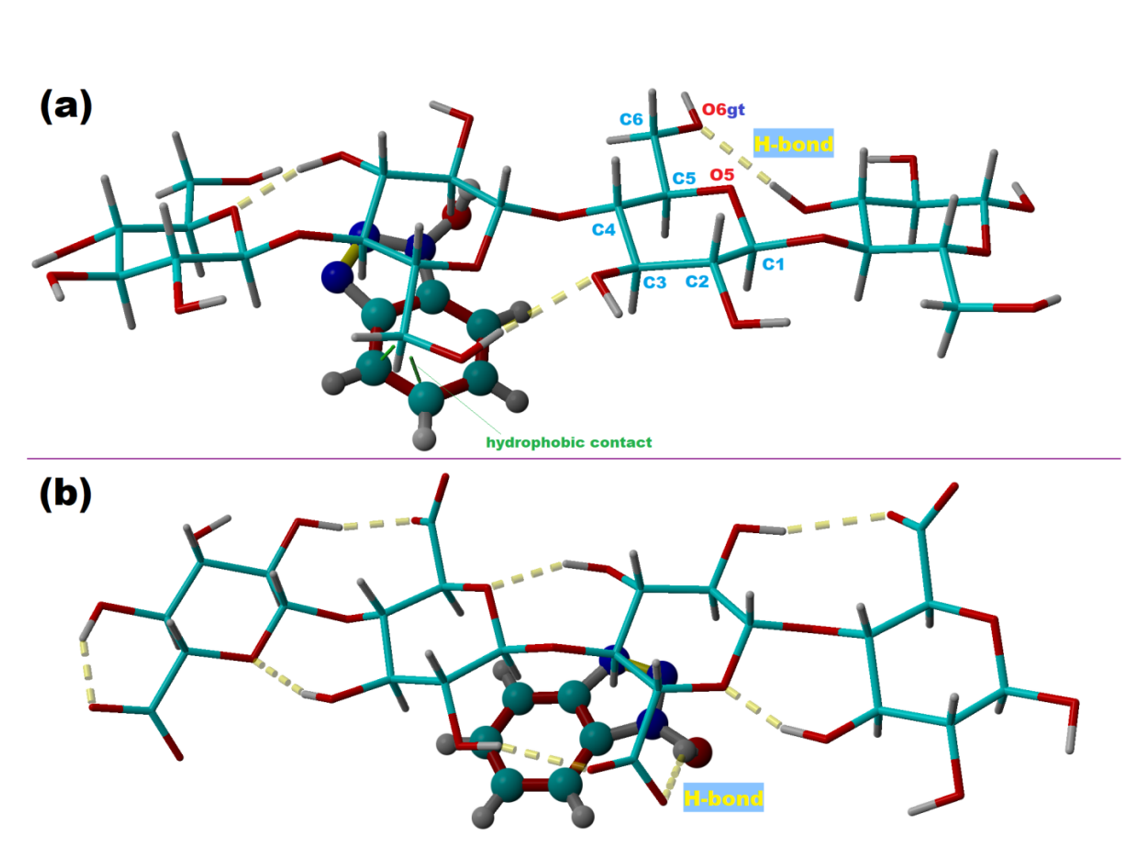
Fig. 3. Molecular docking outcomes highlighting the interaction between cellulose-based tetramers (receptors) and 1-Hydroxybenzotriazole compound (ligand): (a) best docking pose showing the binding mode of the ligand to the cellotetraose receptor; (b) best docking pose showing the binding mode of the ligand to the oxidized-cellotetraose receptor
Cellulose acetate is a cellulose derivative widely used in many applications due to its good solubility in several organic solvents, so it is easily processed and subsequently can be employ for the cellulose regeneration, following a simple deacetylation process under alkaline conditions. Taking advantage of this common process for cellulose regeneration, CA films in DMF were firstly prepared, then cellulose films, which in turn were subjected to a mild oxidation in an oxidizing aqueous solution containing TEMPO, NaClO, and NaBr. Parallel, hybrid films incorporating various amounts of HOBt, following the previously presented route were fabricated, see Figure 4.
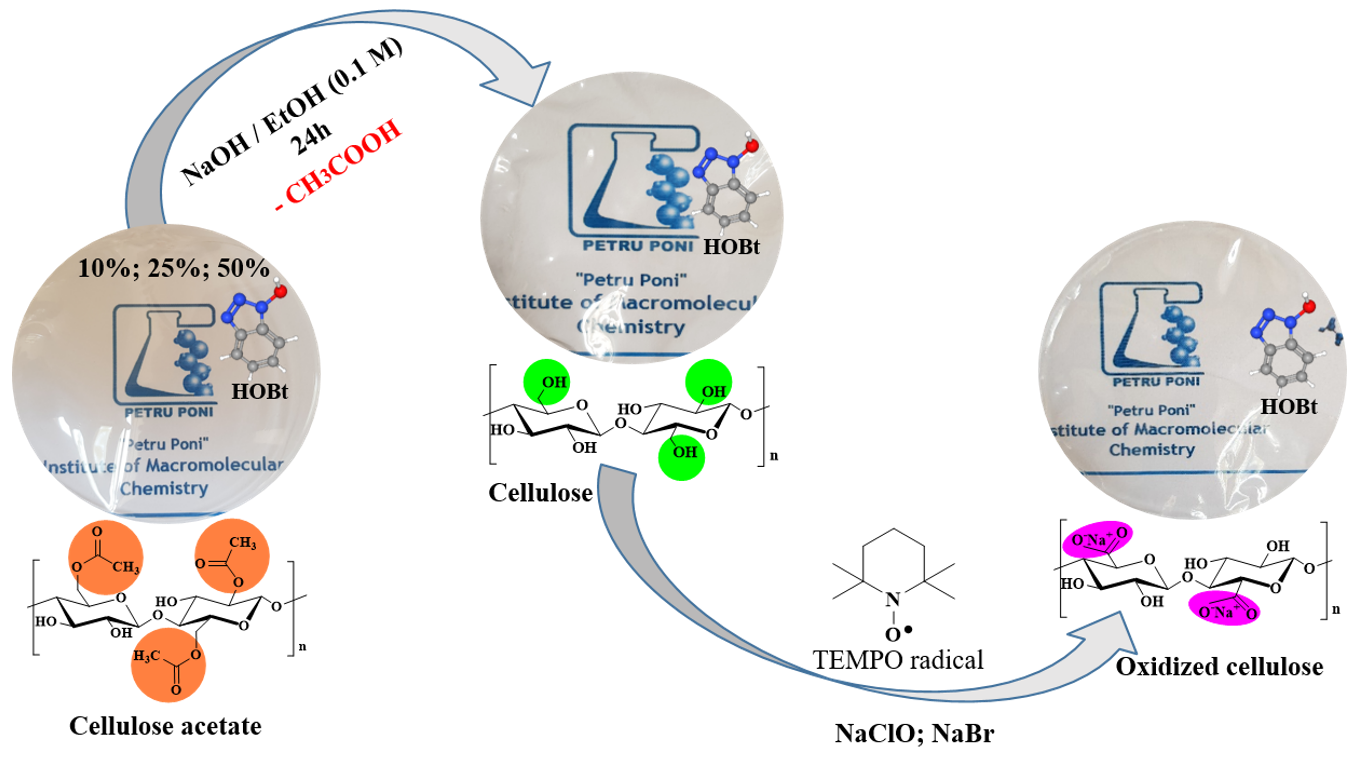
Fig. 4. Schematization of the cellulose acetate films conversion to cellulose and subsequently to oxidized cellulose, and visual appearance of the hybrid membranes made from different cellulosic supports with a initial load of 10%; 25% and 50% (wt.) HOBt
HOBt was chosen to serve as a proton solvent in Nafion membranes, replacing water. The concept of employing nitrogen-bearing heterocycles was first presented some years ago. The benefits of employing such compounds come from their great self-dissociation capacity, which allows them to operate as both a proton donor and acceptor, which is a necessary attribute for good proton conductivity. Furthermore, many heterocycles containing nitrogen have great thermal stability; however, HOBt should be handled carefully, especially when anhydrous, belonging to the category of compounds that, in specific circumstances, pose an explosion danger. However, in prior works in the subject, the authors frequently lamented the inability to introduce a large quantity of heterocycles into the cellulose structure due to its insolubility. Tritt-Goc et al. determined that the greatest achievable level of imidazole in microcrystalline cellulose - imidazole composites corresponds to a molar ratio of 5.0, glucose/imidazole molar weight. By utilizing a cellulose-derivative, such as cellulose acetate, with excellent solubility in organic solvents, it is able to increase the quantity of heterocycle added to the hybrid composite in a systematic and controlled manner. Therefore, the three dosages of HOBt incorporated as a percentage by weight of the CA matrix (10%, 25%, and 50%) resulted in different molar ratios of glucose/HOBt units of 8.1, 3.32, and 1.66, respectively. The protocol was specially designed to determine the impact of the amount of HOBt introduced into the cellulose matrix on the general properties of the resulting films, see Figure 2. Conductometric titration was used to further characterize the oxidized films and establish their level of oxidation following exposure to the oxidizing solution. Thus, it was shown that because the oxidation of cellulose occurs gradually, on the surface of the cellulose film, the addition of HOBt had no effect on the process. All cellulose-HOBt hybrid samples contained roughly 340 ± 18 mmol/kg of carboxylic groups (the median value of three independent experiments), which is comparable to the sample of pure cellulose used as a reference.
In fact, this feature can also be seen by comparing the visual aspect of the films before- and after-oxidation, which remain colorless in contrast to a sample of pure HOBt that under the identical oxidation conditions turns reddish-brown. To assess the possibility of HOBt leaking or washing during regeneration processes, we conducted a series of experiments in which quantities of film with the same thickness (0.025 ± 0.005 mm), accurately weighed using a balance with 10 mg precision, were exposed to the alkaline environment. After 24h, the films were thoroughly cleaned with water, dried, and reweighed. Following these tests, we discovered that the hybrid films do actually lose HOBt after being exposed to an alkaline environment. The actual HOBt content after regeneration was determined to be: 7.8 ± 0.3% (sample C-HOBt10), 21.3 ± 0.6% (sample C-HOBt25), and 41.7 ± 1.6% (sample C-HOBt50). Additionally, the films that had been regenerated and additionally exposed to oxidation were weighed once more, but this time no additional mass losses due to HOBt washing (leakage) were noted. In this way, we can consider that HOBt is not affected under any kind of exposure for 5 min to the oxidizing solution; this only leads to oxidation on the surface of the cellulose. Moreover, using an analyzer equipped with a conventional CHN detector, the regenerate and oxidized samples were also examined in terms of their elemental nitrogen content. The average of three determinations representing the nitrogen content for each sample correlates quite well with the findings from the gravimetric analysis of the films following regeneration and oxidation. The samples of regenerated cellulose therefore had the following nitrogen percentages: 1.96% (sample C-HOBt10), 5.98% (sample C-HOBt25), and 11.68% (sample C-HOBt50). The nitrogen content in the further oxidized films was similar to that in the cellulosic films, as shown in the following examples: 1.925% (Cox-HOBt10 sample), 5.86% (Cox-HOBt25 sample), and 11.55% (Cox-HOBt50 sample) respectively.
EXECUTION STAGE 3/2023
Comprehensive characterization of the physical properties of the fabricated membranes
Films morphology as determined by Scanning Electron Microscopy (SEM) and contact angle (CA)
Scanning electron microscopy was used to examine the morphology of cellulose-based films in fractured cross-section (SEM). The parent films of cellulose acetate have a porous structure, which is seen in the images in Figure 1, and is distributed irregularly in section, with thicker sections alternating with sparse ones. Regenerated cellulose films, on the other hand, seem considerably more regular and consistent, with no significant variances in porosity or pore density, which are properties that oxidized cellulose films retain (Cox).
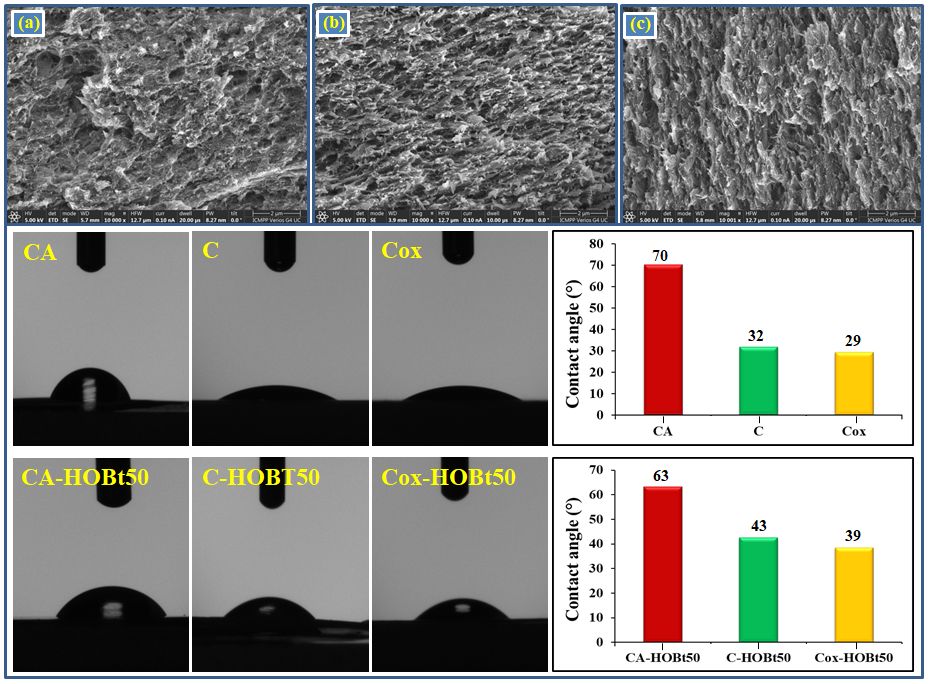
Fig. 1. SEM images of films recorded in fractured cross-section at magnifications 10k of cellulose acetate (a), cellulose (b), and oxidized cellulose (c), along with their contact angle values and comparison with contact angle values of their corresponding composites incorporating 50% (wt.) HOBt (CA-HOBt50 sample), 25% (wt.) HOBt (CA-HOBt25 sample), and 10% (wt.) HOBt (CA-HOBt10 sample), respectivelly
The addition of heterocyclic compound in the polymeric matrix does not have a major effect on its morphology, the structure and porosity being preserved even at the addition of 25% HOBt. However, cellulosic films that incorporate the highest amount of HOBt (C-HOBt50) appear to have an altered morphology, in the sense that their porosity is diminished, while the heterocyclic compound develops a foam like structure.
The measured contact angle values for the prepared films decrease from 70.25o for pure cellulose acetate to 31.74o for cellulose and 29.34o for oxidized cellulose. This decrease of the contact angle confirms that samples C and Cox become more hydrophilic as a result of regeneration and oxidation processes, a feature that is beneficial in PEMs fabrication. When HOBt is added in the films, the contact angle still follows the same trend, but in this case the values are slightly higher than in the case of neat films, Figure 2. The hydrophilic/hydrophobic properties of the films are crucial in ensuring good conductivity. Therefore, in order to evaluate these properties, we determined the swelling degree (%) for both, the starting and hybrid films, see Figure 2.
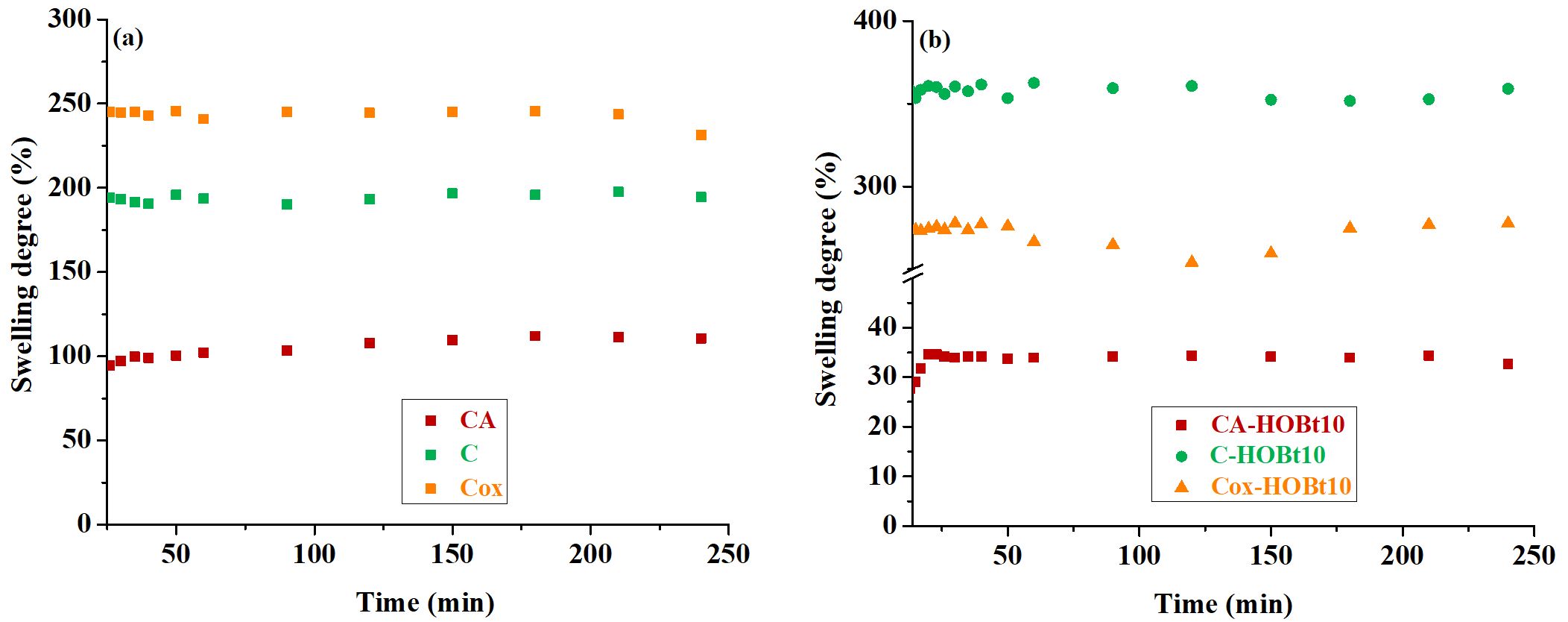
Fig. 2. The swelling degree (%) for the neat (a) and hybrid samples (b) based on CA, C and Cox.
A first observation that can be easily deduced is that cellulose acetate films have a rather low SD (about 100%), which is explicable due to their hydrophobicity, Figure 2a. As expected, cellulose and oxidized cellulose films show high SD values (over 200%), explicable due to their superior hidrophilicity, values that are beneficial for proton conductivity, especially when the vehicle mechanism is involved. Analysing the hybrid samples, i.e. those that incorporate HOBt in the cellulose-based matrix, we found that the content of heterocyclic compound has a negligible influence on SD values, so that only the samples with a minimum amount (7.8 ± 0.3%) of HOBt were shown in Figure 2b. On the other hand, the other two samples, based on regenerated and oxidized cellulose, incorporating 7.8 ± 0.3% HOBt, become even more hydrophilic, with higher SD values than pure samples, especially for the sample C-HOBt10. Thus, while the CA-HOBt10 sample appears to lose even more of its hydrophilic character by introducing HOBt, the other two samples, on the other hand, appear to behave in an opposite manner. These variances result from the various ways that HOBt binds to cellulosic substrates, which vary from one another due to the presence of functional groups that are unique to each substrate.
Dielectric measurements
Due to the reaction conditions, which included an alkaline environment (pH of 10), the oxidized cellulose films were produced as sodium carboxylate. Taking into account that nitro-oxidized carboxylated cellulose nanofibers, both in the form of carboxyl and in the form of carboxylate, have a high efficiency in the preparation of proton exchange fuel cells with high proton conductivity and power density, as well as excellent durability, we chose not to perform additional treatments to subject the films to a hydrochloric acid environment for the conversion of the COONa groups into COOH. The evolution of measured conductivity (σ=ωε”ε0, where ω is the angular frequency, ε” is the measured dielectric loss and ε0 is the vacuum permittivity) and the phase angle (θ(f)=tan-1(Zim/Zre), where Zim and Zre are the imaginary and the real components of the impedance, respectively) as function of frequency and selected temperature values are exemplarily plotted in a common diagram for cellulose membranes with 25% load of HOBt. The analogous isothermal plots of membranes with different HOBt contents revealed similar information. Generally, the measured conductivity may be determined as a sum between the alternating-current conductivity, σac, and the direct-current conductivity, σdc. Following the Figure 3 at low temperatures, the σm(f) profiles exhibit a linear increase and the values of the phase angle are close to -90o. This capacitive behavior is typically for σac component of conductivity and may be assigned with the dipole relaxation phenomena of the polymer backbone. At higher temperatures and low frequencies, a deviation from linear increase of conductivity is observed. As temperature increases, the plateau region of conductivity is considerably developed to higher frequencies while the phase angle is gradually enlarged up to 0o. According to literature, the resistive behavior is attributted to dc-like conduction and origines from the transport of free charge carriers through the polymer medium. We notice that the σ(f) profiles of cellulose acetate membrane with 25% load of HOBt reveals a well-pronounced capacitive behavior with a modest flat region of σdc limited at high temperatures and low frequency (Figure 3a). On the other hand, the resistive behavior seems to dominate in the σ(f) and θ(f) double diagrams of regenerated cellulose (Figure 3b) and oxidized cellulose-based membranes (Figure 3c).
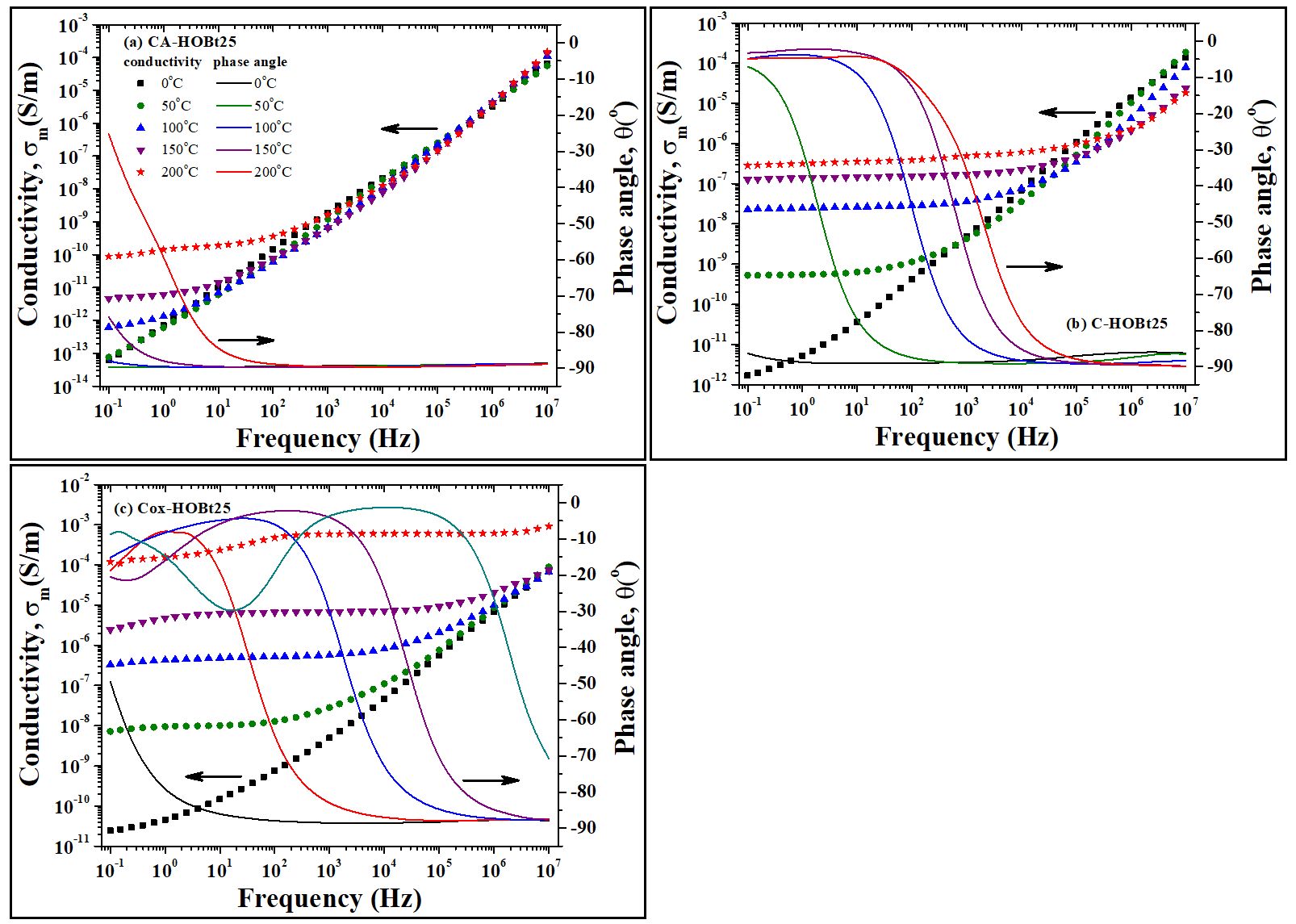
Fig. 3. Representative isothermal plots of measured conductivity (left axis) and phase angle (right axis) as function of frequency at various temperatures for (a) CA-HOBt25, (b) C-HOBt25 and (c) Cox-HOBt25 samples. The legend retrieved in (a) should be also considered for (b) and (c) diagrams
The representative isothermal plots of measured conductivity and phase angle as function of frequency at various temperatures for the unfilled CA, C and Cox samples are presented in the Supporting Information section. In order to evaluate the dc-conductivity of cellulose membranes, the Nyquist plots of the dielectric data were processed with the Cole equation, as reported in our previous work. Essentially, the signal from the Nyquist-type configuration is retrieved as a semicircle, in which, the intersection of the real part of impedance (Z’) with the corresponding imaginary component (Z”) is known to be related to the σdc of the sample. In Figure 4 we represented the typical impedance spectra of cellulose filled with 25% HOBt at various temperatures. The completely achievement of ideal semicircle provided by the Figure 4c, compared with the early stages of development retrieved in Figures 4a and 4b, reveals the highest conductivity for oxidized cellulose-based membrane.
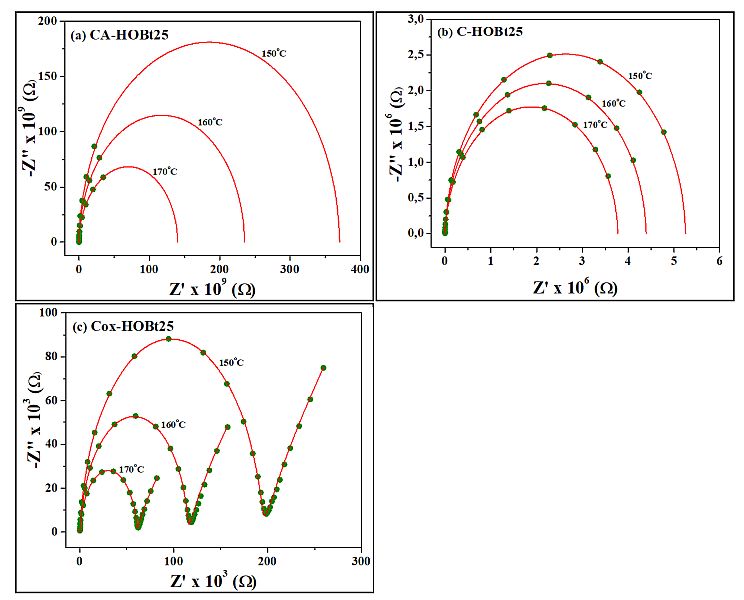
Fig. 4. Representative Nyquist plots of (a) CA-HOBt25, (b) C-HOBt25 and (c) Cox-HOBt25 taken at 150 oC, 160 oC and 170 oC. The experimental dielectric data are represented with solid symbols while the fittings provided by the Cole equation are retrieved with solid lines
The numerical values of dc-conductivity selected at 150 oC for all examined cellulose-based membranes are further presented in Table 1. The insulating nature of matrices is provided by low σdc values, between 10-11 S/m and 10-12 S/m. However, one may observe that the incorporation of HOBt in the cellulose matrix improves notably the conductivity of composite membranes. The effect is obtained since, on the one hand, the HOBt filler is an excellent candidate for protons emitter, and, on the other hand, the particular functional groups of the cellulose matrices favor the transport of protons in these materials. The highest values of σdc are recorded for oxidized cellulose filled with HOBt, suggesting that the functional carboxylic acid groups have a maximal efficiency in promoting the migration of protons through the membrane network. We finally notice that the maximum conductivity of cellulose membranes is almost reached for 10% content of HOBt. Supplementary addition of HOBt leads only to a modest enhance of σdc.
Table 1. Numerical values of σdc for cellulose acetate, regenerated cellulose and oxidized cellulose filled with various contents of HOBt, retrieved at 150 oC
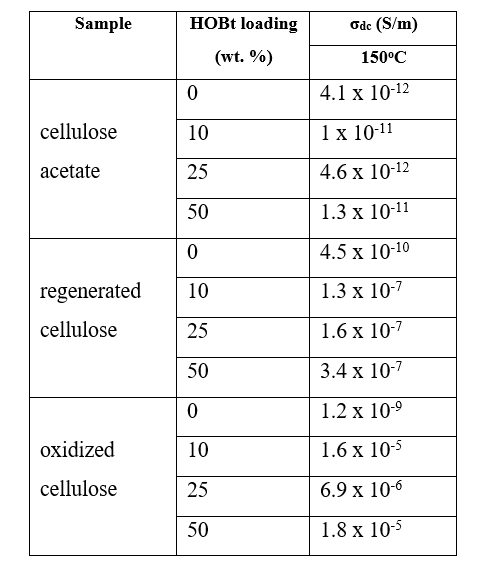
|