|
|
OBJECTIVES 2016:
1. Electro-optical characteristics of polyfluorene with randomly distributed electron-donor and rotaxane electron-acceptor units
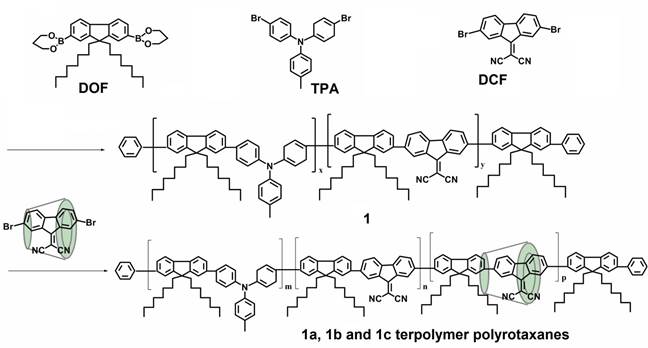
FIGURE 1. Chemical structures of the neat copolymer 1 and 1a, 1b and 1c polyrotaxanes.
In our study, the encapsulation of 9,9-(dicyanomethylene) fluorene (DCF) units into macrocycle cavities was employed with a view to diminish cyano groups quenching effects by solvent, impurities or oxygen molecules. The first step in the preparation of these copolymers is the threading of g-CD, TMS-βCD or TMS-gCD macrocyclic molecules onto DCF (as A units). By reacting DOF (as bulky group) with 4,4`-dibromo-4´´-methyl-triphenylamine (TPA) (as D units) and DCF encapsulated into g-CD, TMS-β-CD or TMS-g-CD in a 5:4:1 molar ratio, followed by the termination of the growing chains with bromobenzene, 1a, 1b and 1c polyrotaxanes were obtained, Fig. 1.
The optical properties of the investigated compounds
The influence of the host nature on the optical properties was investigated by UV-Vis and PL measurements in THF solution or in solid state. The results for the absorption in solid state of polyrotaxanes revealed a strong absorption at about 380,
383 and 385 nm, which can be assigned to the p-p* transition derived from the
conjugated fluorene backbones, Table 1.
Table 1. Optical properties of the investigated compounds
Sample
|
λabs
(nm)
|
λem
(nm)
|
ΦPL
(%)
|
t1
(ns)
|
t2
(ns)
|
DEg
(eV)
|
1
|
383
|
418, 531
|
7.0
|
0.88
|
1.56
|
2.98
|
1a
|
380
|
437
|
19.0
|
-
|
-
|
2.94
|
1b
|
385
|
418, 530
|
17.6
|
1.03
|
-
|
2.88
|
1c
|
383
|
419, 527
|
16.3
|
1.14
|
-
|
2.90
|
The decay traces of the reference 1 obtained from the tF measurements follows a bi-exponential decay consisting of a main component with a relative short time of t1=0.88 (57.08 %) and a minor component with a longer lifetime of t2=1.56 ns (42.92 %), Table 1. The observed behavior would suggest that the bi-exponential decay of the non-rotaxane 1 can be associated to the intrachain emission and excitonic lifetime, whereas in the polyrotaxanes only to the excitonic contribution.
Electrochemical properties
CVs of these investigated compounds are shown in Figure 2. CV scans of the samples exhibit the oxidation and reduction processes. From their onset potentials, it was possible to estimate precisely the values of HOMO (IP), LUMO (EA) and DEg. The observed CVs behaviors suggest that the encapsulation of DCF moieties results in a smaller electrochemical DEg, HOMO, as well as LUMO than that of the non-rotaxane counterpart.
FIGURE 2. CV of the investigated polymeric films coated on Pt plate electrodes in 0.1 M TBAPF6)/ACN solution at a scan rate of 20 mV·s-1
The HOMO/LUMO energy levels of the unthreaded polymer 1 and its corresponding 1a polyrotaxane in combination with the electronic potentials of the anodic indium tin oxide (ITO) glass substrate (-4.75 eV) and cathodic aluminum (-
2.2 eV), prove that the required energy level is fulfilled for fabrication of organic light-emitting diodes (OLEDs). The HOMO/LUMO energy levels of 1b and 1c in combination with the work function of anodic ITO glass substrates coated with poly(3,4-ethylenedioxythiophene):poly(styrenesulfonate) (PEDOT:PSS) (-5.2 eV) and cathodic Ca (-2.8 eV) or Al (-2.2 eV) indicate that they are also electrochemically accessible as electron-transporting materials. The relative position of the frontier energy levels of such architectures may also be advantageous to photovoltaic (PV) applications. Furthermore, these results suggest that the polyrotaxanes could be useful for the fabrication of diffuse hetero- junctions between interpenetrating networks of n- and p-type semiconductors. In addition, the encapsulation of the conjugated polymer units into TMS-βCD or TMS-
gCD cavities leads to distinct improvements of solubility of resulting polyrotaxanes
in common organic solvents (e.g., THF, DCM, CHCl3 or toluene) with respect to 1a, which is soluble in THF/DMF = 3/1 v/v. Also, with encapsulation of per-TMS modified CDs, the transparency of solid films has been improved along with the increased molecular weights and glass-transition temperatures. Overall, the HOMO/LUMO energy levels of studied polyrotaxanes proved that these polyrotaxane structures, as well as their references are electrochemically accessible as electron-transporting materials.
2. Synthesis of polyfluorenes with persylilated β- and g-cyclodextrin main-chain polyrotaxanes
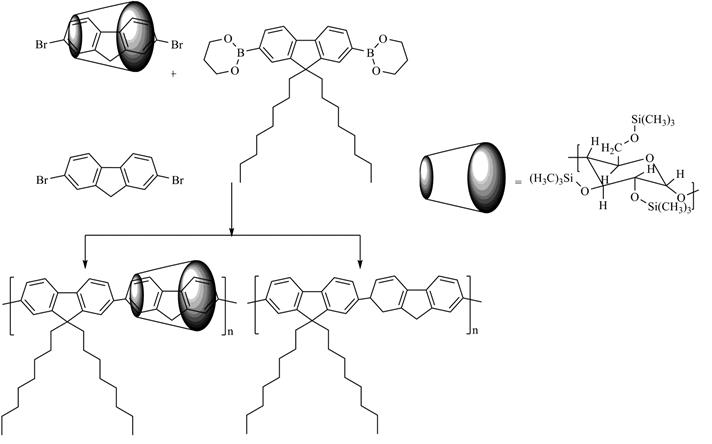
SCHEME 1. Synthesis of polyfluorenes/persylilated β- and g-cyclodextrin main-chain polyrotaxanes
In our study, the encapsulation of 9,9-(dicyanomethylene) fluorene (DCF) units into macrocycle cavities was employed with a view to diminish cyano groups quenching effects by solvent, impurities or oxygen molecules. The first step in the preparation of these copolymers is the threading of g-CD, TMS-βCD or TMS-gCD macrocyclic molecules onto DCF (as A units). By reacting DOF (as bulky group) with 4,4`-dibromo-4´´-methyl-triphenylamine (TPA) (as D units) and DCF encapsulated into g-CD, TMS-β-CD or TMS-g-CD in a 5:4:1 molar ratio, followed by the termination of the growing chains with bromobenzene, 1a, 1b and 1c polyrotaxanes were obtained, Fig. 1.
TMS-CD encapsulation of PFs backbones leads to distinct improvements in the solubility, molecular weights, film forming ability and thermal stability. The optical investigations confirmed that the polyrotaxanes exhibited higher fluorescence quantum yield and fluorescence lifetimes.
Polyfluorenes/persylilated β- and g-cyclodextrin main-chain polyrotaxanes have been synthesized through the Suzuki cross-coupling reaction of 2,7- dibromofluorene encapsulated into TMS-βCD, TMS-gCD or CB7 cavities and 9,9-
dioctylfluorene as bulky stopper units, Scheme 1. In this approach a small excess of
9,9-dioctylfluorene was added at the end of polymerization to introduce ester groups at both polymer chain ends, and finally bromobenzene was added as a monofunctional end-capping reagent to react with the boronic ester end groups. Thus, a copolymer having stable phenyl groups at both ends was obtained. The spatial distribution of octyl groups is larger enough than the diameter of the inner cavities of TMS-βCD, TMS-gCD or CB7 indicating their blocking effect. Encapsulation of 2,7-dibromofluorene units into macrocycle cavities has been used to protect its 9 position against oxidative degradation. 1H NMR spectrocopy provides a low coverage value for the polyrotaxane derived from TMS-gCD compared to those obtained from TMS-βCD. The formation of PF polyrotaxanes also was confirmed by SEC analysis, where no free macrocycle peaks was present in the chromatogram, which clearly denoted that the polyrotaxane samples are not a physical mixture between components. The chromatogram of polyrotaxanes is situated at lower elution volumes as compared to that corresponding to the non-threaded copolymers, evidencing their higher molecular weights. The presence of TMS-βCD or TMS-gCD onto PF backbones increase the thermal stability of main-chain polyrotaxanes and improves their solubility in halogenated solvents.
The UV-Vis absorption spectra of the non-rotaxane shows a featureless band peaking at 374 nm. Upon encapsulation with the TMS-βCD, a hypsochromic shift of about 7 nm attributed to a reduction of intermolecular interactions and/or a variation of the polarity, when the PF core is inside the macrocycles cavity is identified. The PL emission of the non-rotaxane copolymer shows three vibronic components at about 418,
435 and 460 nm. The intensity of the 0-1 PL band (435 nm) for diluted CHCl3 solution is the most intense. At the same concentration, PF polyrotaxane with TMS-βCD exhibited a slight blue-shift (2 nm) of the emission. The ratio of the emission intensity of the 0-0 transition for this polyrotaxane is higher than that of the 0-1 transition, contrary to what we observe for the non-rotaxane counterpart. Such trends suggest that the encapsulation with TMS-βCD acts to reduce intermolecular interactions, in agreement with previous reported results. It appears that TMS-βCD is much more effective than TMS-gCD for suppressing intermolecular interactions upon an increase of the polymer concentration. The temporal decays of 10-3 mg×mL-1 in CHCl3 solutions of the polyrotaxane with TMS-βCD and the reference are mono-exponential with a τF of ~ 0.6
ns, whereas the polyrotaxane with TMS-gCD shows a bi-exponential decay consisting of
a main component with τF1 = 0.7 ns (98%) and a minor component with a longer τF2 =
5.4 ns (2%). The longer τF for the polyrotaxane with is TMS-gCD, consistent with
interchain states and they are plausible, considering the significantly bigger size of the
gCD, which might favor both unthreading of the cores, or even accommodation of more than one core unit within the macrocycles cavities. The HOMO/LUMO energy levels of these polyrotaxane copolymers imply that all copolymers are electrochemically
accessible as electron-transporting materials.
Cucurbit[7]uril based fluorene polyrotaxanes
Poly[2,7-(9,9-dioctylfluorene)-alt-(2,7-fluorene/cucurbit[7]uril)] polyrotaxane was synthesized according to Suzuki coupling protocol in DMSO, by reacting 2,7- dibromofluorene encapsulated into the cucurbit[7]uril (CB7) cavity with 9,9- dioctylfluorene-2,7-diboronic acid bis(1,3-propanediol) ester. The Ka value (9.5 ×103 M–1) indicates that 2,7-dibromofluorene provides a preferential binding to CB7, which allows
the synthesis of a polyrotaxane with CB7/structural unit ratio of about 1/3 and higher molecular weights. The chemical structure was proved by FTIR and 1H-NMR spectroscopy. The resulting polyrotaxane with CB7 contains 27.4% coverage (quantified by 1H-NMR), but some error is expected due to its low solubility in DMSO-d6. Note that DMSO-d6 it is not a good solvent for PFs chains and that only CB7 induces polyrotaxane`s solubility. The 1H-NMR in DMSO-d6 shows characteristic peaks for both PFs chains and CB7, but the signals are not very well resolved. The thermal, optical, electrochemical, wetting and surface morphological properties of the polyrotaxane have been investigated and compared to those of the neat copolymer. The polyrotaxane exhibits an enhancement in the glass transition temperature, blue-shifts absorption of about 11 nm and a subtle effect on the LUMO energy levels. The fluorescence lifetime follow a mono-exponential decay with a value of tF = 0.7 ns. The electrochemical band gap of the polyrotaxane (3.39 eV) is smaller than that of the neat compound. The comparison of the HOMO/LUMO energy levels of the polyrotaxane and the corresponding non-rotaxane with the electronic potentials of the anodic indium tin oxide (ITO) glass substrate (−4.75 eV) and cathodic aluminum (−2.2 eV) reveals that the required energy level criteria for the fabrication of organic light-emitting diodes (OLEDs) are nicely met.
Despite the advances made by these preliminarily investigations, additional information on energy levels of charge carriers in terms of polyfluorene polyrotaxanes in solid states, the nature of electrolyte and solvent, and electrodes in electrochemical doping/dedoping processes require further exploration. The broad range of properties outlined here can be tuned by straightforward structural modifications designed to make them suitable alternate replacements for the non-rotaxane counterparts, especially if one takes into account of their good fluorescence efficiency, surface morphology, high oxidative and chemical stability, and quenching strength. The macrocyclic encapsulations by various classes of macrocyclic molecules suppress the tendency of aggregate formations by diminishing intermolecular interactions of PF backbones. The properties of PF polyrotaxanes can be readily optimized by incorporation of various host molecules, such as CB7. The presence of CB7 results in improved solubility in water, which allows their processing by spin-coating. Development of new polyrotaxane architectures should be beneficial, especially in the field of materials for the generation of active layer in organic electronic devices, such as organic light emitting diode (OLED) and photovoltaic (PV) devices. These findings are relevant to their applications in OLED, where it is essential to prevent luminescence quenching without hindering charge transport. It is believed that further research into conjugated polyrotaxanes for organic electronics will continue to furnish excellent achievements in succession to the rapid progress. Despite the interest in synthetic approaches, the interdisciplinary collaborations serve to continue a high level of interest for the generation of active layer in organic electronic devices.
|
|

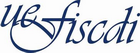
|